Recently, Victor Black (@victorblackmasterclass) made a post on Instagram touting that “DHT as shown here is a most capable Anabolic Agent”. With “as shown here” referring to a trial by Idan et al. in which the long-term effects of dihydrotestosterone (DHT) treatment on prostate growth in healthy middle-aged (~60 yr old) men were evaluated [1]. His statement is based on the fact that the men receiving DHT gained 1.5 kg of LBM over the course of 2 years (of which 1.4 kg was gained at the 6-month mark). According to Victor Black, this would refute my claim that DHT’s effect in muscle is dramatically diminished by its breakdown there. And, by extension, also my claim that methenolone’s (Primobolan) effect in muscle is diminished by its breakdown there, as extensively covered in a previous article of mine.
However, if anything, the study perfectly supports my position that DHT’s effect is strongly diminished in muscle. In this article I will explain how these results demonstrate this. In a previous article I’ve already narrated out the reason why this is the case. Briefly: DHT is susceptible to degradation by 3α/3β-hydroxysteroid dehydrogenases (3α/3β-HSD). 3α-HSD demonstrates high activity in skeletal muscle, and, as a result, DHT is rapidly broken down in this tissue [2]. An old German paper elegantly demonstrated this by intravenously injecting labeled DHT to three participants in order to track its metabolic fate [3]. Muscle tissue samples were collected 20 to 60 minutes after injection. 29.4–51.0 % of the labeled steroid was identified as 3α-androstanediol (the 3α-reduced metabolite of DHT) and 7.2-31.2% as androsterone (also a 3α-reduced metabolite) in these samples. A couple of other metabolites were also identified and only 18.1 to 26.2 % of DHT was left. This indeed demonstrates a significant breakdown of DHT occuring in skeletal muscle tissue. As a side note, they did the same thing with labeled testosterone, of which 69.5–79.8 % of the labeled steroid was still identified as testosterone in the muscle tissue samples.
This is gonna be a long and technical article, but it’s necessary to explain everything thorough and properly. Let’s dive into the study at hand.
DHT is a very potent androgen
First some background knowledge. DHT is a very potent androgen. Physiologically speaking, it’s the most potent naturally occurring androgen in humans [4]. Clinically, this is evidenced by severely hampered male sexual development at birth and during puberty in patients with 5α-reductase deficiency. For sufficient androgenic action during male sexual development, testosterone is required to be converted to DHT in tissues (richly) expressing 5a-reductase. In adulthood, however, the conversion to DHT does not appear to be mandatory to sustain sufficient androgenic action in most, if not all, tissues [4]. As long as testosterone levels are normal, testosterone exerts enough androgenic action on its own.
A ‘ceiling effect’ seems to be present for certain androgenic actions. That is, beyond a certain serum testosterone concentration, there isn't any additive effect. This is, for example, the case for the trophic effect on the prostate at physiological testosterone concentrations (~8–30 nmol/L) [5, 6]. Addition of the potent 5α-reductase inhibitor dutasteride had no effect on prostate volume in healthy men aged 18-50 years when, after chemical castration, receiving roughly half a testosterone replacement therapy (TRT) dosage (50 mg testosterone enanthate weekly) [5].
Some androgenic actions, however, are clearly amplified by conversion to DHT in the adult male. For example, patients with 5α-reductase deficiency do not develop male-pattern baldness in adulthood [7]. Indeed, pharmaceutical inhibition of 5α-reductase serves as a good treatment modality for this condition [8]. As such, it’s evident that physiological testosterone concentrations aren’t hitting a ‘ceiling effect’ in this case and conversion to DHT can contribute to an androgenic effect.
Clearly, there doesn’t appear to be a ceiling effect anywhere close to the physiological range of testosterone levels with regard to muscle-building as well. Nevertheless, the conversion of testosterone to DHT doesn’t amplify this effect. This is supported by the fact that 5α-reduction inhibition doesn’t affect changes in fat-free mass in response to graded testosterone doses up to 600 mg testosterone enanthate weekly [5]. Moreover, patients with 5α-reductase deficiency undergo normal muscle development during pubertal transition [9]. However, there appears to be no detectable 5α-reductase activity in human skeletal muscle [10]. As such, skeletal muscle would need to rely on DHT coming from the circulation. Since serum DHT concentrations are very low compared to testosterone’s (0.47–2.65 nmol/L [11]), and as mentioned above, it gets broken down extensively in muscle mass, DHT is indeed unlikely to contribute to muscle-building under physiological conditions.
Mechanistically, it’s widely agreed that DHT’s stronger androgenic action, at least in part, is due to DHT’s higher binding affinity for, and lower dissociation rate from, the androgen receptor compared to testosterone [12]. Indeed, this is also reflected in an androgen receptor bioassay conducted in human osteosarcoma cells, which demonstrates a roughly five-fold higher androgen receptor activation for DHT compared to testosterone with equimolar concentrations [13]. Or, in other words, five molecules of testosterone led to similar androgen receptor activation as one molecule of dihydrotestosterone in this assay. Each molecule of DHT is roughly five times as potent as testosterone in activating the androgen receptor. Of note: osteosarcoma cells do not express any enzymes to break down DHT or testosterone to a significant degree. Effects of tissue-specific metabolism thus aren’t reflected in it.
The DHT dosage in the study was high
The subjects in the trial by Idan et al. received 70 mg of transdermal DHT daily, which led to a steady-state nadir serum concentration of 23 nmol/L. Baseline testosterone levels of the men in this trial were 15 nmol/L. The DHT dosage is unmistakably a high dosage, given that: 1) it exerts a several-fold stronger androgenic action than testosterone at equimolar concentrations (as explained above), and 2) it resulted in serum levels 31% higher than baseline testosterone levels. But let’s see how this androgenic action holds up in skeletal muscle, where it gets broken down.
A pharmacokinetics trial employing the same DHT preparation, but at a slightly lower dosage (64 mg daily), also in healthy elderly men, almost perfectly matches the measured serum concentration in the trial by Idan et al. [14]. So I’m gonna use some of this data. Since serum concentrations fluctuate (with the nadir concentration being the lowest one over time, i.e. before the next application of a drug), a snapshot at a given moment in time doesn’t tell the whole story. A better picture can be obtained by looking at the area under the curve (AUC) value. Mathematically speaking, this is the integral of the serum concentration over time. This concept is a lot clearer when visualized. It’s simply the grey striped surface area below the serum concentration curve:
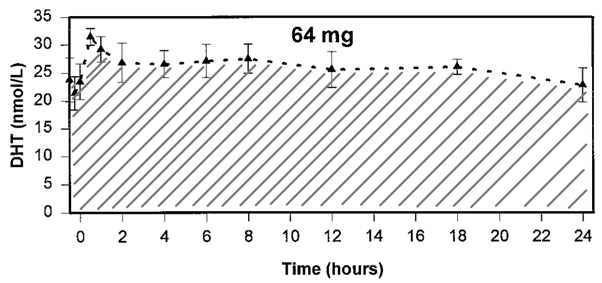
The AUC is thus a measure of the exposure to a compound over time. In this case, the AUC over 24 hours was ~625 nmol h/L. Additionally, while testosterone was greatly suppressed, there was still some remaining endogenous testosterone which was good for an AUC over 24 hours of ~100 nmol h/L.
Why all this nerd stuff? After all, the DHT concentration doesn’t fluctuate much, so looking at the AUC doesn’t add much, right? However, it does fluctuate quite a bit if you’d look at the testosterone concentration after weekly injection of testosterone enanthate. In order to make a fair comparison—since I’m gonna compare the two—I figured it would be best to take the AUC into account of each, instead of the nadir serum concentration. As such, we can make a nice comparison with a trial, also in healthy older men, in which testosterone was administered in various dosages [15]. We thus can draw a comparison between the two based on the androgen exposure over time to determine how potent DHT is compared to testosterone, molecule per molecule, in terms of its muscle-building effect. To make the comparison slightly more fair, I’ve picked the results at the 6-month mark rather than at the 2-year mark, since the other trials lasted (a lot) shorter anyhow. Which would likely put them at a disadvantage as there would be less time for the anabolic actions to take place.
Below I’ve summarized the effect of exogenous testosterone, DHT, and two other AAS [16, 17], on lean body mass (LBM) in healthy older men, as well as some other useful data to make meaningful comparisons. (I’m reporting fat free mass (FFM) as LBM, as the two are virtually the same.) I’ve calculated the AUC for the testosterone trial assuming a steady-state half-life of 7 days for intramuscular testosterone injections [18]. Since testosterone injections were given weekly, I’ve provided the AUC over a period of a week (i.e. 168 hours).
And, to make the comparison even more fair, I’ve calculated the free concentration of testosterone and DHT to also include the free fraction AUC (fAUC). After all, DHT binds more strongly to SHBG than does testosterone, and as such its free concentration is lower for any given concentration. If I wouldn’t correct for this, the results would be biased towards testosterone, and I just want an honest presentation of the facts. Anyways. For this purpose, I’ve used the Vermeulen equation [19]. Firstly, I've assumed an association constant of SHBG of 1.6 x 109 M-1 and 5.5 x 109 M-1 for testosterone and DHT, respectively [20]. I've also assumed an association constant of albumin of 3.6 x 104 M-1 and an albumin concentration of 4.3 g/dL for both [19]. (And, of course, I’ve taken the SHBG concentrations from the studies themselves.)
Parameter | 50 mg TE weekly | 125 mg TE weekly | 70 mg DHT daily | 20 mg OXA daily | 50 mg OXY daily |
---|---|---|---|---|---|
Mean age (years) | 66 | 67 | 61 | 73 | 71 |
Nadir serum levels (nmol/L) | 9.5 | 29.5 | 23 | - | - |
168 h AUC (nmol h/L) | 2,303 | 7,150 | 4,375 | - | - |
168 h fAUC (nmol h/L) | 29.5 | 132.4 | 35.4 | - | - |
LBM (kg) | +1.7 | +4.2 | +1.4 | +3.0 | +3.3 |
FM (kg) | -0.9 | -1.5 | -0.9 | -1.9 | -2.6 |
LH (IU/L) | N.A. | N.A. | -68 % | -40 % | -71 % |
LBM and fat mass (FM) were determined by DEXA scans in all of these trials. As you can see, the increase in LBM and the decrease in FM was about the same for 50 mg testosterone enanthate weekly and 70 mg transdermal DHT daily. The men in the testosterone trial were chemically castrated to exclude the effect of any endogenous testosterone. As such, half a testosterone replacement therapy dosage was as potent as 70 mg transdermal DHT daily for building muscle and decreasing fat mass in healthy older men. Total DHT exposure over time was 90% higher than that of testosterone. (If you’d add the remaining testosterone exposure on top of that of DHT, this would be a 120% difference.) If you look at the free fraction AUC, DHT exposure was still 20% higher than that of testosterone in the testosterone trial. (Again, not even taking the remaining testosterone concentration into account.) This is a relatively small difference and for simplicity you could view the molecule per molecule exposure as being roughly similar.
While DHT wasn’t potent at muscle building, it surely was potent at suppressing LH levels. With a nonaromatizing androgen, this is indicative of a high dosage. LH levels decreased to a similar degree as with administration of 50 mg oxymetholone daily (which led to roughly twice the LBM gains and FM losses), and a seemingly stronger degree than 20 mg oxandrolone daily (which also demonstrated more potent muscle-building and fat-loss properties).
Additionally, clear androgenic effects were the cause of withdrawal from the study in those treated with DHT as the following passage highlights: “A total of 13 men in the DHT group and 5 in the placebo group discontinued treatment. Men who had protocol-specific discontinuation were all in the DHT group (8 had polycythemia and 3 had an increased PSA level 4.0 g/mL). “ (There were a total of 56 men in the DHT group and 58 in the placebo group.)
Conclusion
DHT is a very potent androgen. The best estimate in the literature is that it’s roughly five times as potent as testosterone in terms of activating the androgen receptor. If my claim that DHT’s androgenic action in skeletal muscle is severely diminished by its breakdown there is false, a far greater muscle-building effect would be expected when comparing an equimolar exposure of the androgen compared to testosterone. However, with similar equimolar exposure, DHT results in a similar muscle-building effect as testosterone. As such, its several-fold stronger potency in terms of androgen receptor activation is completely nullified in muscle tissue by its breakdown there.
In androgen-sensitive tissues which don’t display a “ceiling effect” at physiological testosterone concentrations, and don’t breakdown DHT extensively, DHT’s androgenic action will, of course, be pretty damn high. Or, in other words, this will lead to relatively more side effects arising from these tissues.
(I was unable to find pharmacokinetics studies of oxandrolone and oxymetholone which included AUC values. I’d be interested in adding this data to the table as well for comparison. If anybody reading this knows such a study, please contact me.)
References
- Idan, Amanda, et al. "Long-term effects of dihydrotestosterone treatment on prostate growth in healthy, middle-aged men without prostate disease: a randomized, placebo-controlled trial." Annals of internal medicine 153.10 (2010): 621-632.
- Kicman, Andrew T. "Pharmacology of anabolic steroids." British journal of pharmacology 154.3 (2008): 502-521.
- Becker, H., et al. "In vivo uptake and metabolism of 3H-testosterone and 3H-5α-dihydrotestosterone by human benign prostatic hypertrophy." European Journal of Endocrinology 71.3 (1972): 589-599.
- Marchetti, Paula M., and Julian H. Barth. "Clinical biochemistry of dihydrotestosterone." Annals of clinical biochemistry 50.2 (2013): 95-107.
- Bhasin, Shalender, et al. "Effect of testosterone supplementation with and without a dual 5α-reductase inhibitor on fat-free mass in men with suppressed testosterone production: a randomized controlled trial." Jama 307.9 (2012): 931-939.
- Morgentaler, Abraham, and Abdulmaged M. Traish. "Shifting the paradigm of testosterone and prostate cancer: the saturation model and the limits of androgen-dependent growth." European urology 55.2 (2009): 310-321.
- Imperato-McGinley, Jullianne, and Y-S. Zhu. "Androgens and male physiology the syndrome of 5α-reductase-2 deficiency." Molecular and cellular endocrinology 198.1-2 (2002): 51-59.
- Gupta, Aditya K., and Andrew Charrette. "The efficacy and safety of 5α-reductase inhibitors in androgenetic alopecia: a network meta-analysis and benefit–risk assessment of finasteride and dutasteride." Journal of dermatological treatment 25.2 (2014): 156-161.
- Imperato-McGinley, Julianne, et al. "Steroid 5α-reductase deficiency in man: an inherited form of male pseudohermaphroditism." Science 186.4170 (1974): 1213-1215.
- Thigpen, Anice E., et al. "Tissue distribution and ontogeny of steroid 5 alpha-reductase isozyme expression." The Journal of clinical investigation 92.2 (1993): 903-910.
- Shiraishi, Steve, et al. "Simultaneous measurement of serum testosterone and dihydrotestosterone by liquid chromatography–tandem mass spectrometry." Clinical chemistry 54.11 (2008): 1855-1863.
- Swerdloff, Ronald S., et al. "Dihydrotestosterone: biochemistry, physiology, and clinical implications of elevated blood levels." Endocrine reviews 38.3 (2017): 220-254.
- Houtman, Corine J., et al. "Detection of anabolic androgenic steroid abuse in doping control using mammalian reporter gene bioassays." Analytica chimica acta 637.1-2 (2009): 247-258.
- Wang, C., et al. "Comparative pharmacokinetics of three doses of percutaneous dihydrotestosterone gel in healthy elderly men–a clinical research center study." The Journal of Clinical Endocrinology & Metabolism 83.8 (1998): 2749-2757.
- Bhasin, Shalender, et al. "Older men are as responsive as young men to the anabolic effects of graded doses of testosterone on the skeletal muscle." The Journal of Clinical Endocrinology & Metabolism 90.2 (2005): 678-688.
- Schroeder, E. Todd, et al. "Treatment with oxandrolone and the durability of effects in older men." Journal of Applied Physiology (2004).
- Schroeder, E. Todd, et al. "Effects of an oral androgen on muscle and metabolism in older, community-dwelling men." American Journal of Physiology-Endocrinology and Metabolism 284.1 (2003): E120-E128.Schroeder, E. Todd, et al. "Effects of an oral androgen on muscle and metabolism in older, community-dwelling men." American Journal of Physiology-Endocrinology and Metabolism 284.1 (2003): E120-E128.
- Kaminetsky, Jed, Jonathan S. Jaffe, and Ronald S. Swerdloff. "Pharmacokinetic profile of subcutaneous testosterone enanthate delivered via a novel, prefilled single‐use autoinjector: a phase ii study." Sexual medicine 3.4 (2015): 269-279.
- Vermeulen, Alex, Lieve Verdonck, and Jean M. Kaufman. "A critical evaluation of simple methods for the estimation of free testosterone in serum." The Journal of Clinical Endocrinology & Metabolism 84.10 (1999): 3666-3672.
- Dunn, James F., Bruce C. Nisula, and David Rodbard. "Transport of steroid hormones: binding of 21 endogenous steroids to both testosterone-binding globulin and corticosteroid-binding globulin in human plasma." The Journal of Clinical Endocrinology & Metabolism 53.1 (1981): 58-68.